
Using this method, Thomson soon identified the positive ions from H 2, H and He.
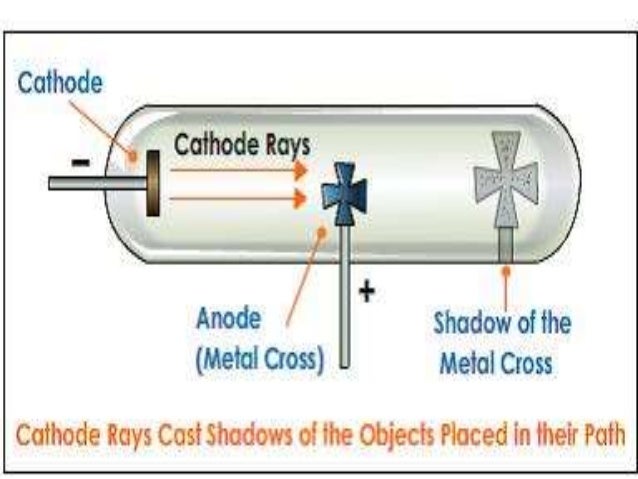
The net effect is that they will record as portions of a parabola, with a separate parabola for each e/ m species present. Thus in reality, species with a single e/ m value will have a dependency on v 2 in the x direction and v in the y direction. 6 The positive rays, however, will comprise particles with a range of velocities. Simple manipulation of equations ( i) and ( ii) eliminates v, the velocity term, from the equation.Įquation ( iii) implies that a particle with a specific e/ m value (or m/ e) will have a specific position on the xy screen plane, and another particle with a different e/ m value (but holding B and E constant) will record in a different position. The deflection in the y-direction depends on the magnetic field ( B), and along the x-axis by the electrical field ( E). Later variations used photographic films to record on, thus giving a permanent record of the experiments. Coated with a material that fluoresced when hit by the rays, it enabled the deflections induced by the electrical and magnetic fields to be recorded more accurately. Thomson decided to work, essentially, with Wien's apparatus, but with one important difference: he allowed the positive rays to impinge on an oscilloscope-like screen at the end of the tube. From the results I have already got, it is clear that m/ e for the positive ions produced by an incandescent wire must be at least 1000 times the value of negative ions, and this is only the inferior limit. I am engaged with some experiments on the effect of the magnetic field on the convection of electricity by positive ions. the carriers of positive electricity at low pressures seem to be ordinary molecules, whilst the carriers of negative electricity are very much smaller. (He) has measured the ratio m/ e for positive ions in a (discharge) tube and found that it is the same order as the value of m/ e in ordinary electrolysis. 4 Presumably, Willi was working with residual air in his tube, a mixture of nitrogen and oxygen (28 and 32 amu, respectively).įollowing on from Willi Wien's discovery, Thomson published a paper at the end of 1899, revealing his interest in positive rays. Interestingly, 100 years later, Wien's relative, Karl Wien, working at the Darmstadt Institute for Nuclear Physics, reworked the figures in modern units and obtained the m/ e ratio as 3.2 × 10 -7 kg/C, which corresponds to a value of 30 amu, assuming singly charged particles. 3 He deduced (but does not give a calculation to show how) that the rays consist of a particle beam with m/ e ratio of 3.2 × 10 -3 'CGS units'. Using a magnetic field of 3250 'CGS units' (probably gauss) and a electrical field of 2000 V, applied in the same direction of the magnetic field, Wien's positive ray showed a deflection of 6 mm. Using very powerful electromagnets, he observed that the canal rays deflected in a direction opposite to the cathode rays, which implied they carried a positive charge of electricity, and this led them to be renamed as 'positive rays'. One year later, in 1898, Wilhem (Willi) Wien, while working as an assistant to Hermann Helmholtz in Berlin, was inspired by Thomson's work and began his own investigations into cathode and canal rays. Source: © National Library of Congress/SPL Using the strongest magnet he had, one that certainly had an effect on the cathode rays, Goldstein attempted to deflect his canal rays, but he observed no change in path. 2a Unlike the cathode rays, the colour of the canal rays depended on the identity of the residual gas in the tube, though the significance of this observation was not appreciated at the time. On account of their appearance, Goldstein named them Kanalstrahlen, which means 'canal rays'. If the cathode was relatively thick, they would appear as a parallel beam. Some years later, in 1886, the German physicist Eugen Goldstein noticed that if he used a perforated cathode, then in addition to the cathode rays between the anode and the cathode, there was a different type of ray emerging from the holes in the cathode, and moving in the opposite direction to the cathode rays. 1 This was early evidence of 'cathode rays', though nothing was known of their properties. In 1858, professor of physics at the University of Bonn, Julius Plücker, while investigating the action of a magnet on the electric discharge of rarefied gases, observed a cathode-induced green fluorescence on the glass walls of a discharge tube. The phenomenon began to receive rigorous scientific study in the mid-19th century with the advent of induction coils that could provide high voltages sustained over long periods of time and the availability of high capacity batteries to power the induction coils.
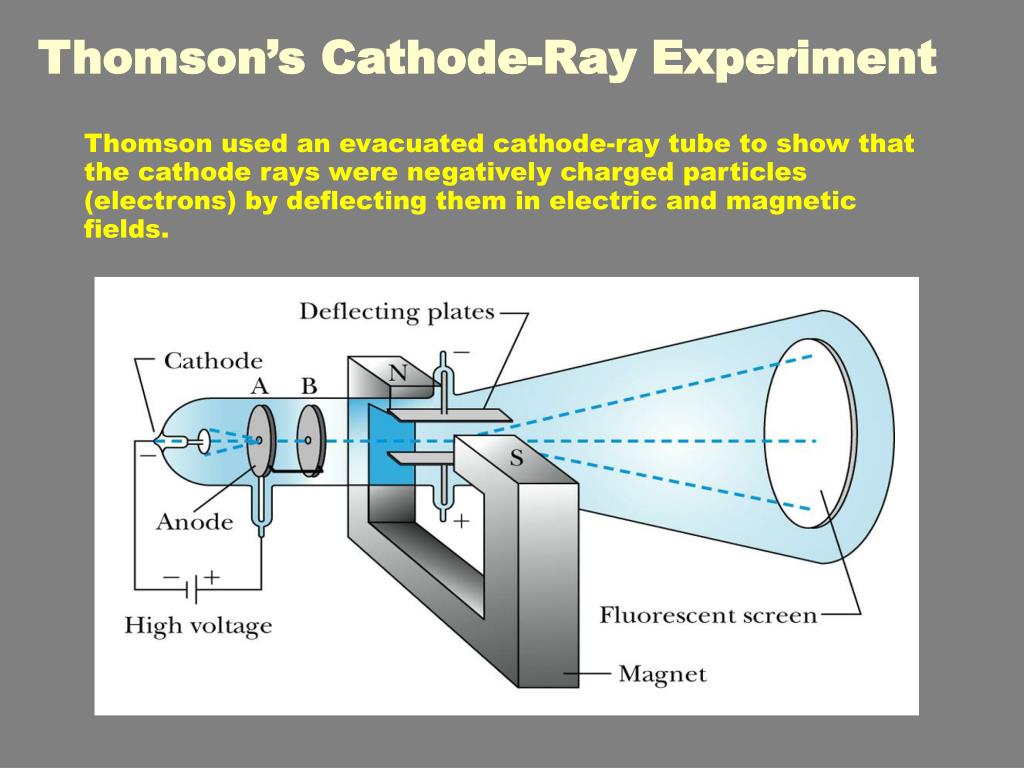
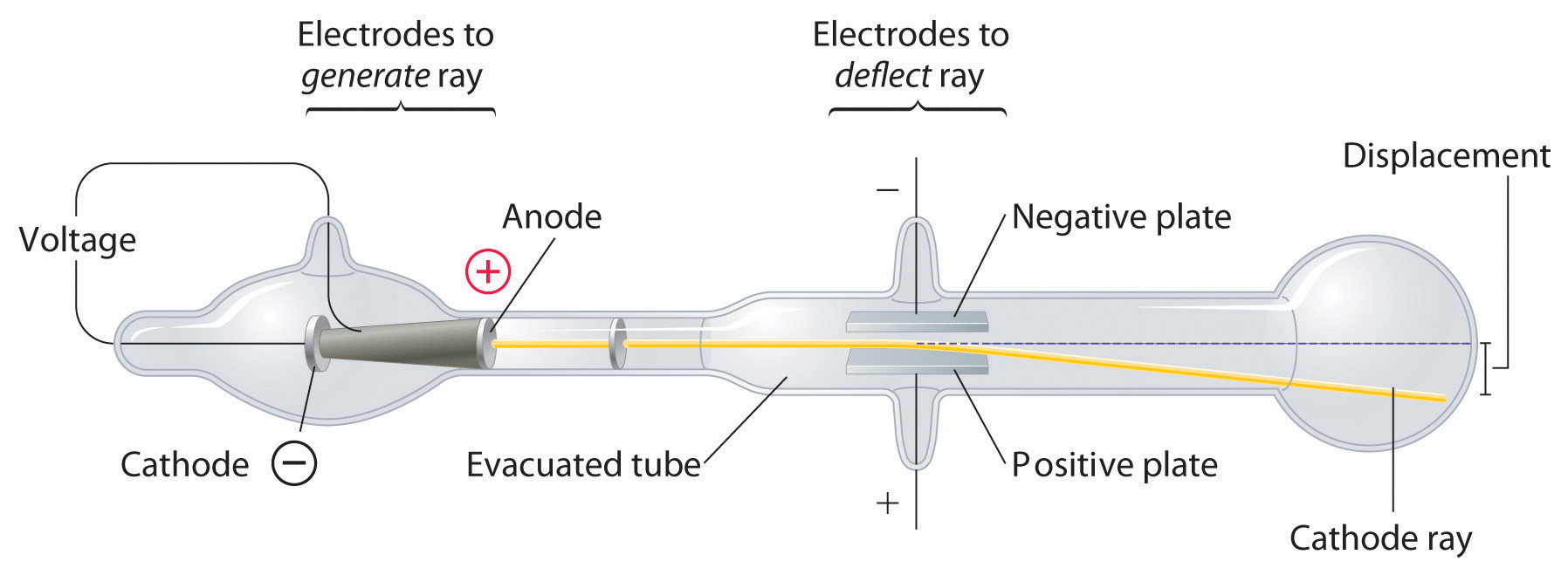
At the end of the 18th century experimental scientists were aware that a spark generated electrostatically could travel longer distances in a partially evacuated glass tube than in air.
